Veldhorst Lab
Menno Veldhorst (Harderwijk, 1984) is the QuTech Academy lead. Veldhorst received his PhD-degree cum laude in 2012 for his research on superconducting and topological hybrids at the university of Twente and was awarded the PhD Overijssel award (see also). He then moved to the university of New South Wales in Sydney to work on quantum computation using silicon quantum dots. Highlights were the demonstration of quantum operations on a single qubit and quantum operations between two qubits. These two together represented the first demonstration of universal quantum logic in silicon, an important step towards a silicon quantum computer, and announced by Physics World as one the top ten breakthroughs in physics in 2015.
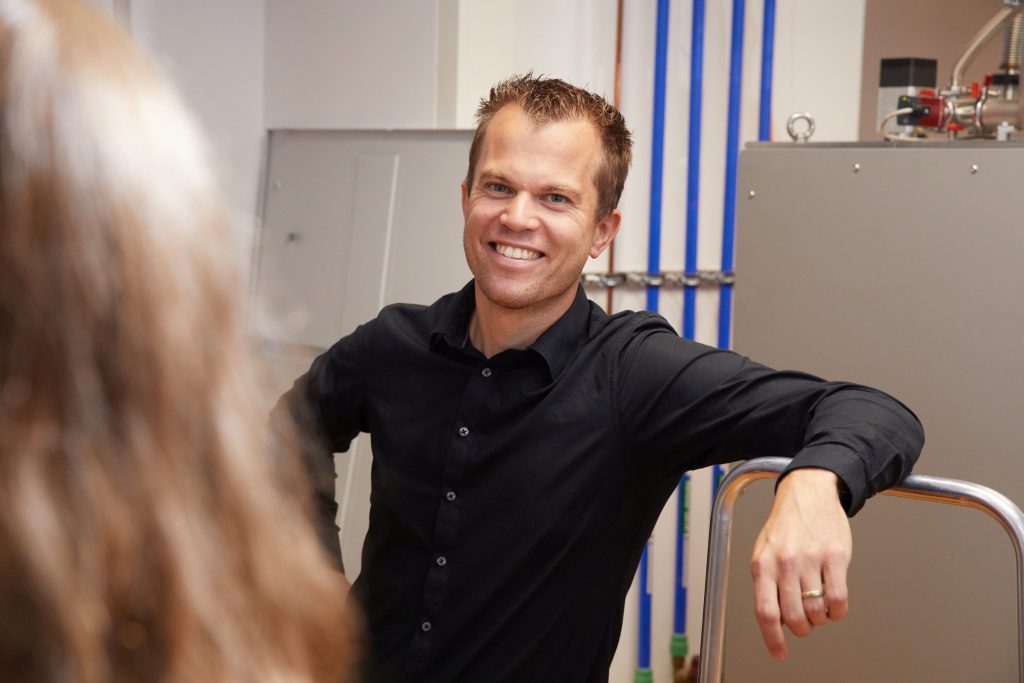
The goal of Veldhorst lab is to engineer emergent quantum phenomena in a tunable fashion, to simulate non-trivial quantum physics, and to compute classically hard problems. We do this by confining individual electrons and holes in gate-defined quantum dots and coherently controlling their spin states.
The development of quantum physics over the last century has been pivotal in our understanding of chemical interactions, the electronic wave functions in semiconductors, and the propagation of light. This has led to an enormous amount of devices and applications, such as lasers for telecommunication and transistors for computing. The remarkable development defined the basis for the information age and it is sometimes dubbed as the first quantum revolution. Nonetheless, as impressive as supercomputers are today, they are insufficient to simulate many processes occurring in nature. Quantum systems can quickly yield a complexity that is impossible to describe using instruments that obey classical physics laws, since quantum mechanical interactions can lead to a complexity that scales exponentially with its size. This motivated Richard Feynman to state: ‘Nature isn’t classical, dammit, and if you want to make a simulation of nature, you’d better make it quantum mechanical, and by golly it’s a wonderful problem, because it doesn’t look so easy’. Fortunately, advances in the field of nanotechnology over the last few decades has enabled to individually control particles such as electrons. Rather than understanding and appreciating the relevance of quantum physics, it is now becoming feasible to use its most profound and fundamental concepts, such as superposition and entanglement, to build revolutionary technology. The rise of quantum technology constructed by actively involving the quantum mechanical nature of individual particles is called the second quantum revolution. Our group aims to build such quantum technology using the same advanced semiconductor technology that enabled the construction of billions of transistors on a single silicon chip, offering a scalable system where the relevant parameters can be controlled at will. We are motivated by the opportunity to study the deepest aspects of quantum mechanics, while having the chance to seeing our research being materialized in real-life applications through collaborations with industrial giants. The long-term ambition of Veldhorst lab is therefore to engineer emergent quantum phenomena in a tunable fashion, to simulate non-trivial quantum physics, and to compute classically hard problems.
We start from electrons confined in quantum dots built out of group IV materials. The spin states of a single electron define the prototypical qubit and it can maintain quantum coherence over human timescales when using group IV semiconductors as host material [Veldhorst Nature Nanotechnology 2014]. Furthermore, electrons confined in quantum dots can be controlled and coupled to each other with great precision [Veldhorst et al Nature 2015]. This provides all the ingredients for a universal quantum gate set, which our group demonstrated in silicon [Petit et al. Nature 2020] and in germanium [Hendrickx et al. Nature 2020] and up to comparatively high temperatures above one Kelvin. Moving forward, we showed that quantum dot qubits can be positioned in a grid [Lawrie et al. APL 2020, van Riggelen et al. APL 2020]. We used this capability to realize 4-qubit logic [Hendrickx et al. Nature 2021] and demonstrated the coherent generation of an entangled 4-qubit GHZ state, the most advanced demonstration with quantum dots today and highly relevant for quantum simulation and quantum error correction. Single electrons and holes can also be coupled to macroscopic states such as superconductivity. In a preliminary experiment we showed gate-tunable superconducting proximity effects in germanium [Hendrickx et al. Nature Comm. 2018] and over length scales of several micrometers [Hendrickx et al. PRB 2019].
Enabled by these achievements, we can now implement quantum algorithms and study quantum simulation. Simultaneously, there are many exciting fundamental physics questions that need to be resolved. These activities define Veldhorst lab as a multi-disciplinary group and we collaborate with many groups around the world to pursue our ambitions.
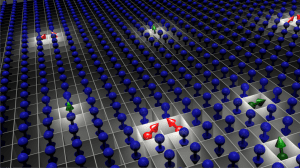
Artist impression of a silicon quantum computer performing single- and two-qubit operations
Are you interested to join Veldhorst lab, please feel free to contact me.
Contact information:
e-mail: m.veldhorst@tudelft.nl