Algorithmic tuning, feedback and calibration in spin qubit arrays
As qubit device complexity grows, even for small NISQ processors and especially for gate-tuned quantum devices as described in the previous section, tuning and controlling the entire system becomes prohibitively expensive. The need to calibrate individual qubits in such arrays, either to maximize fidelity initially or when parameters drift, is a complicated and repetitive process, requiring deep understanding of cross-talk, correlations, and physics-informed design of algorithms.
We work on algorithm-aided tuning, readout, feedback, and control, focusing on small-scale 2D spin qubit arrays; for instance, the automated stabilization of the qubit array over time against slow sensor or environmental drifts. Even for a small number of qubits, this could mean the difference between a working small-scale simulator and an inoperable system due to the prohibitive cost of drift compensation. We use methods such as Bayesian optimization, evolutionary strategies, and physics-informed neural networks. For readout, we work on novel signal processing techniques that accurately and in real time (implemented on-FPGA with tens of nanosecond latency) can classify the digitized waves to infer a multi-qubit state from a resonator response.
This work is supported by the EU Research in Action program (IGNITE project) and the US Army Research Office (AUTOBAHN project).
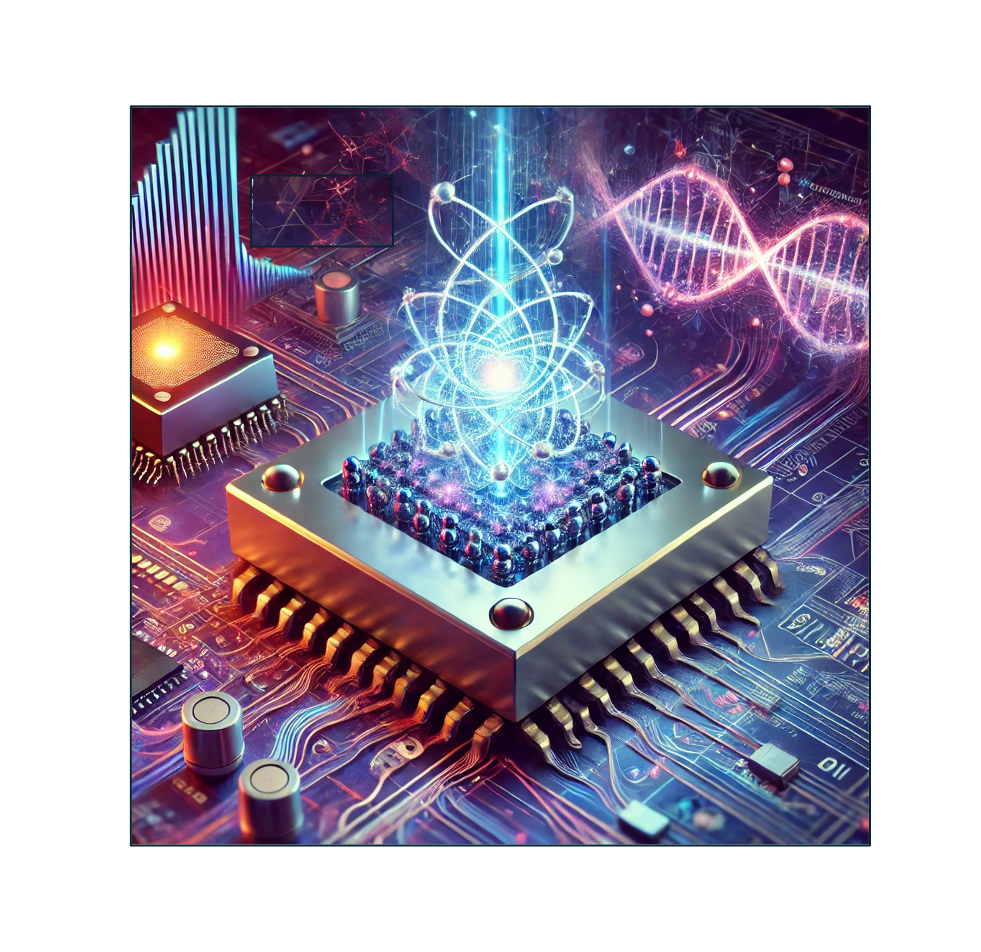
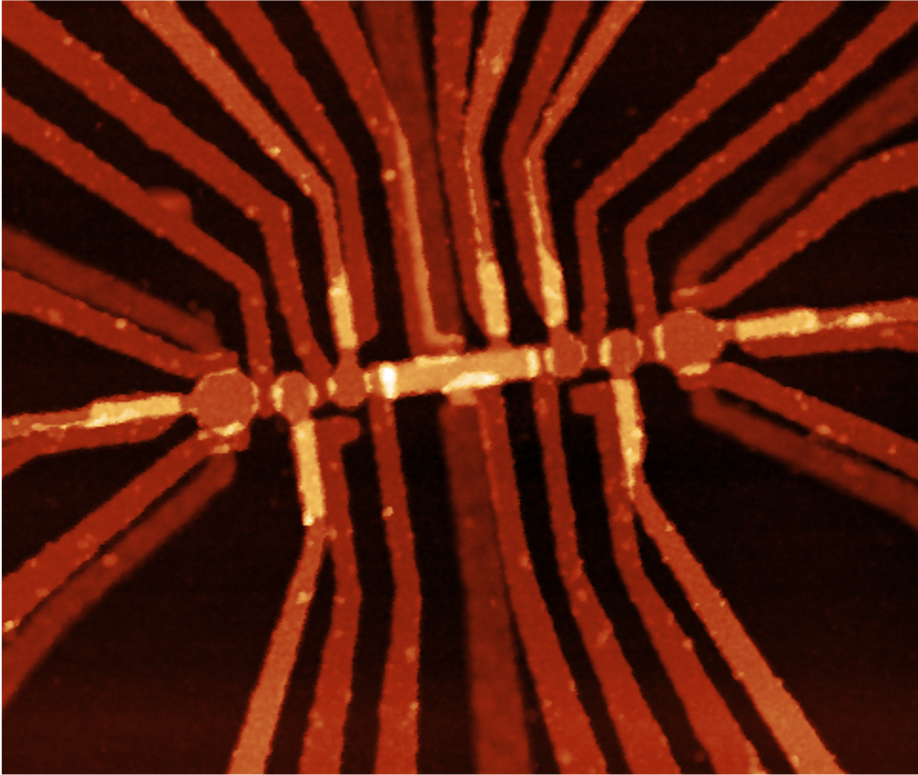
Germanium superconductor-semiconductor hybrid circuit
Like our research group, germanium is a material that rests at the quantum computation-condensed matter interface. Ge heterostructures can host hybrid superconductor-semiconductor devices with novel materials-science methods allowing for transparent superconducting contacts. Germanium can also be isotopically purified, a process that led to record coherence times for silicon. Germanium also possesses the ease of fabrication of GaAs but does not suffer from piezoelectricity, which makes it attractive for integrating superconducting resonators with spin qubits and Andreev bound states, for better readout and novel physics. Currently, my group is developing devices incorporating small (2×3) spin qubit arrays coupled via proximitization-induced crossed Andreev reflection (CAR), as well as exploring the integration of superconducting resonators with this material. This could lead to the development of new parametric amplifiers, protected superconductor-semiconductor qubit modalities, or complex phase-transitions in engineered super-semi Josephson junction arrays.
This work is supported by the EU Research in Action program (IGNITE project), NWO covenant funding, and the EU Pathfinder program (ELEQUANT project).
Quantum-dot and superconducting resonator circuits
Superconducting resonators coupled to semiconductor spins, could help study the transfer of energy between vibronic modes and artificial atoms. Quantum dots and dot arrays, display discrete electronic states similar to quantum states of atoms and molecules. Connecting superconducting resonators to specific points in these arrays could controllably affecting the tunnel coupling, the chemical potential, or the spin state in a particular spatial or energy configuration. The effect of the resonator photon population on shell structure and the dot array’s degeneracy breaking may help us understand frustration in ordered quantum dot lattices, biochemical energy transfer processes, light-matter interaction and many-body optics.
Engineering the low temperature connections to, and measurement of, these complex devices involves specialised wiring, sample holders and carefully engineered materials which have excellent transmission at low temperature. We are working with Delft Circuits, start-up company with specialised expertise in developing cryogenic wiring solutions, to ensure we can measure and control these devices. This project is financially supported by Holland High Tech (TKI) project 24PPS025. The four-year project started on July 1st, 2024.
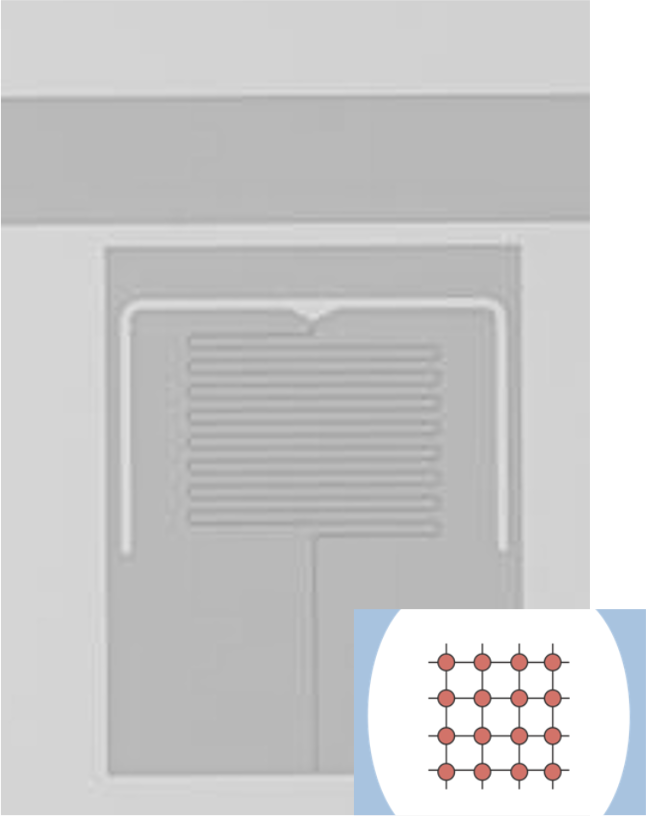