Stories of Quantum
It all comes together
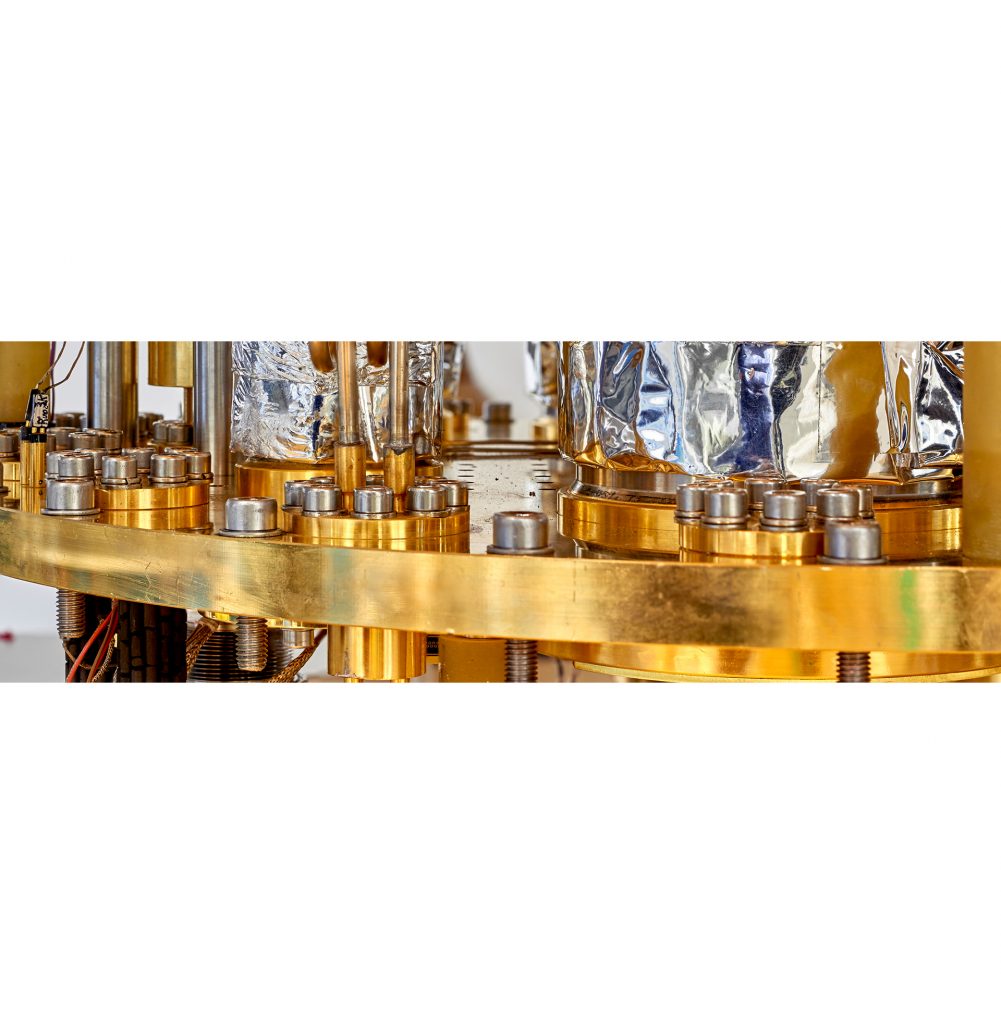
It all comes together
Interview with Menno Veldhorst
Menno Veldhorst is group leader at QuTech and roadmap leader of the QuTech Academy. The story on how he got here almost seems pre-planned. However, the truth appears to be very different.
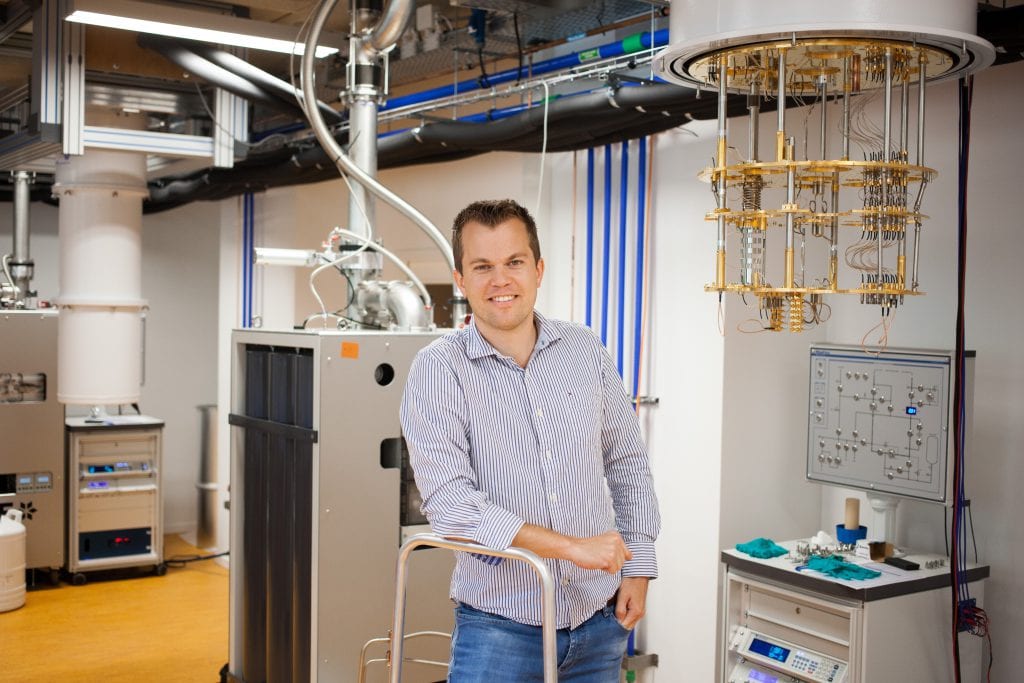
While pursuing his PhD in Twente, Veldhorst focused on superconductivity in topological hybrids. This was a subject involving relatively simple experiments, but a lot of complicated theoretical work, which earned him the Overijssel PhD Award in 2013. After this success, he moved to Australia. Here, he worked in a completely different field at the University of New South Wales, studying silicon (a material that has already been the subject of a great deal of research). This gave him the opportunity to carry out complex experiments. From there, the step to QuTech was a logical one, he says: “At QuTech I can combine the knowledge I gained in my PhD with executing advanced experiments, to make further progress in the exciting field of quantum information.”
It’s not as if his career path had been planned from the start, Veldhorst explains: “As far as my career is concerned, I am not a long-term planner. I’m just very curious, so if I succeed at something I am keen to know how far I can take it. At the same time I’m not easily satisfied. When I feel I have thoroughly understood something I seek something new: taking a step to broaden my horizon or taking a step sideways. I think that’s a better way of looking at my career: considering not just content, but also how my role changes. As a postdoc I was mainly trying to get my head around new ideas, while in my current role I have to consider whether I can convey ideas to my group as well. These are completely different aspects to my work that I have to focus on now, and it’s precisely these changes that draw me to my work so much.” In addition to being group leader, Veldhorst is also roadmap leader at QuTech Academy: “The more aspects my work entails, the more focused I become.”
He doesn’t feel it’s necessary to map out a route towards an ideal situation. “I might have to be careful with the advice I give to students, for whom I think it’s very good to think about their career path. However, I also feel it’s very good to be able to deviate from it. You have to dare to make choices that bring uncertainty with them. Often, if the preconditions are right, you can steer and define a position along the way. It’s good not to get stuck on a question such as, ‘Is this the perfect place?’ No, instead, make that place perfect for you.”
Persistence is also very apparent in Veldhorst’s research. When he worked on silicon qubits in Australia, many people thought this would never be possible. However, the team succeeded and Physics World named their publication in Nature as one of the ten most important physics breakthroughs in 2015. Veldhorst is still working on removing obstacles to realising a practical quantum chip: “The road to a quantum computer is like a big puzzle and I love putting the right pieces together in order to solve the puzzle. It’s even better if people think it won’t work, this motivates us even more to prove them wrong. ‘No’ is not an option for me.”
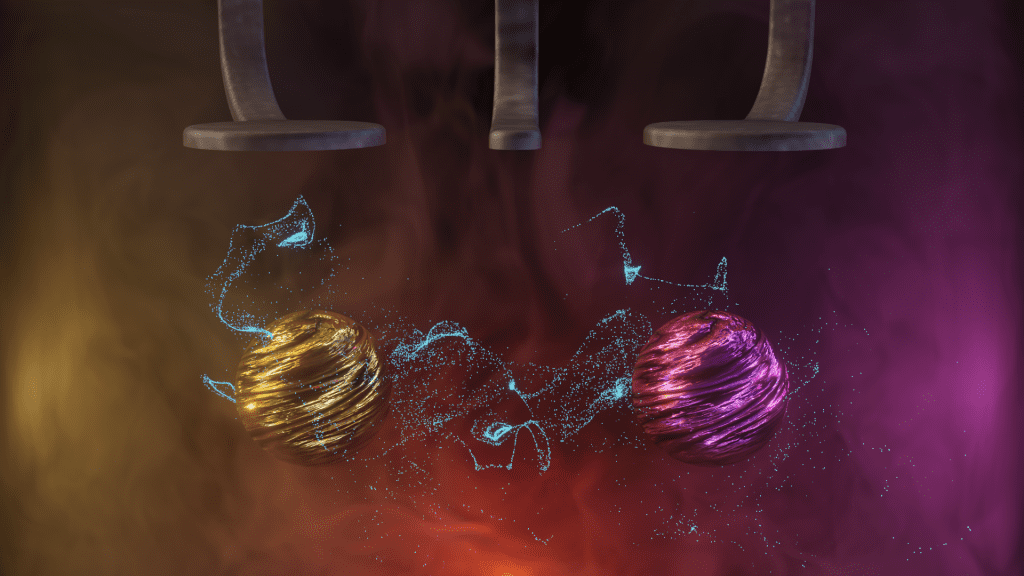
Artist impression of two entangled spin qubits. The group of Veldhorst showed two-qubit operations in germanium and silicon, and in silicon even at temperatures above one Kelvin. Credit: Luca Petit.
Scaling up the number of qubits, for example, remains a challenge: it is not yet possible to make a large number of qubits work together. A logical approach is to use qubits that can be made using production methods of classic chips, such as superconductor or semiconductor qubits. However, this is not a straightforward solution, because at the moment researchers must often counteract the undesirable influences of the qubits by operating the qubits at ever-lower temperatures. These temperatures are difficult to achieve when dealing with the millions of qubits needed for a practical quantum computer. Veldhorst is also working on this challenge: his group has published a study in Nature showing that silicon qubits can also operate at temperatures above one Kelvin. “This temperature is of course still very low, but it is already high enough for integrating the qubits and other electronics onto the same chip”, according to Veldhorst.
A short time before, his group published another study in Nature on the use of germanium (rather than silicon) for the production of semiconductor qubits. Germanium is the material used for the very first transistor in 1947, and it shows a physical effect called spin-orbit coupling. Other qubits need external elements to control them, such as magnets or electrical wires and this is another factor that complicates the scaling-up. Veldhorst explains: “Germanium quantum dots have all the essentials: these structures are made with the same technique as the transistors we currently use as a building block for classical computers, and we can make billions of them on a chip. We imagine that with germanium, qubits can be scaled up in the same way.”
“It all comes together in my research on germanium: many of the physics concepts that I worked with during my PhD are also applicable to that material. This allows me to link those two focus areas in my career: on the one hand we understand germanium very well, and we can experiment a lot with it, but on the other hand there are also many new things to explore further. We can bring all the elements together in a very controlled way, which allows us to explore new methods. For example, we can research whether there are other, better methods to make qubits. It is a material that lends itself well for the discovery of new opportunities in quantum technology, which I find very exciting.”