21.02.2025Qubit Research
Integrating a semiconducting quantum dot with a superconductor
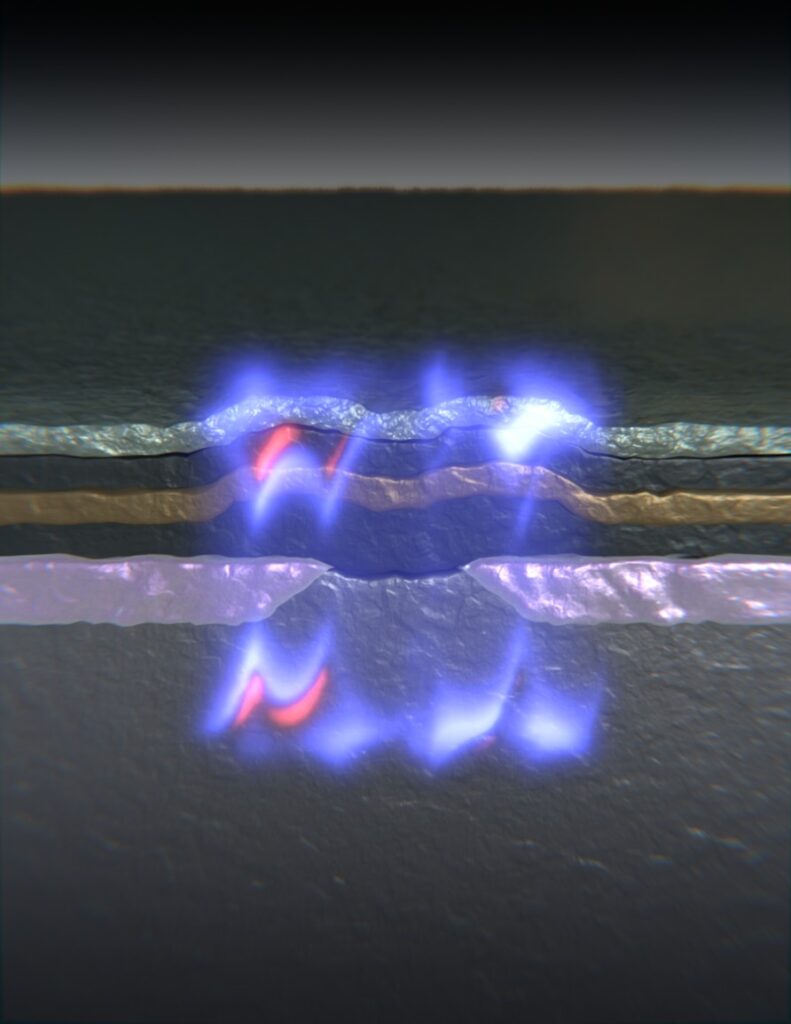
An international research team from QuTech and the University of Copenhagen has developed a superconductor-semiconductor hybrid device. It contains a quantum dot in germanium proximized by a superconductor. The integration of on-demand superconductivity into a semiconductor enables the potential development of new hybrid quantum processors. The researchers published their results in Nature Materials.
Both semiconductor and superconductor materials host leading qubit implementations, each with their own advantages. However, superconductors have proved hard to integrate into semiconductors that host coherent spin qubits, and these respective qubits are therefore usually implemented in separate platforms and considered separate fields. Now, researchers have cracked the puzzle on how to combine these two different materials onto one platform.
Combining benefits within a single architecture
Semiconducting and superconducting materials each have their respective benefits when hosting qubits for quantum technology. Semiconducting spin qubits are created by trapping single electrons or holes in a controlled semiconductor structure. They have proven to be excellent candidates for building a large-scale processor on a single chip, given their compact size and compatibility with industrial semiconductor manufacturing. Superconducting qubits on the other hand can be considered as current loops in a superconductor, going one way or another. Superconducting circuits can span millimeter distances, and therefore connect spin qubits over larger distances (as shown in a recent QuTech breakthrough).
For this study, researchers led by Anasua Chatterjee at QuTech, in collaboration with the group of Ferdinand Kuemmeth from the University of Copenhagen, developed a device combining both materials onto a single platform. For this, they incorporated a superconducting material in a germanium semiconducting heterostructure, which hosted a quantum dot.
Chatterjee explains: “Using lithography methods, we were able to pattern superconducting areas precisely where we wanted them to be in our device. We showed that they formed a coherent bound state with trapped charges in the semiconductor. This is really encouraging for the emerging field of hybrid superconductor-semiconductor circuits.”
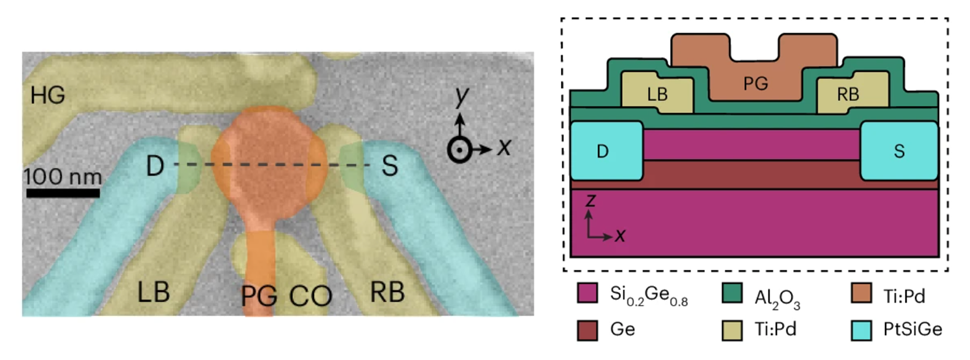
Device image from the top, with a cross section on the right showing how the researchers incorporated superconductivity (by creating the PtGeSi material, in blue). Other electrodes (RB for example) control the interaction between the superconducting and semiconducting parts of the device.
New materials and new techniques
Previously, researchers attempted to combine superconductors and semiconductors by growing alternating layers of different materials on top of each other. To subsequently pattern the semiconductor, parts of the material were etched away. This approach risked damaging the delicate superconductor-semiconductor interface, degrading its quality.
Chatterjee and her group managed to fabricate their device using a different technique, which was developed in the group of QuTech group Leader Giordano Scappucci. His group discovered a process which involves patterning platinum on top of a grown semiconductor heterostructure. By sufficiently heating this structure, the platinum formed a platinum germanosilicide -a superconducting polycrystalline lead- with the heterostructure underneath in the exact patterned design.
Scappucci explains: “Two years ago we developed a method for integrating superconducting polycrystalline germanides with germanium semiconductor devices using a simple thermally-driven solid state reaction. The method is robust and has been replicated by other experimental teams at IBM and at Copenhagen. Now in the experiment led by Chatterjee all these building blocks come together into a complex quantum dot device, which opens new directions for industry-compatible quantum technology.”
A major result is the showcasing of superconductivity in germanium and coherent bound states using this new, less-invasive method. Germanium, like silicon, is a material from group-IV of the periodic table, compatible with the CMOS industry and an excellent host for spin-based qubits, as shown previously by the group of study co-author Menno Veldhorst. Nuclear spins, which are a major decoherence path, can be removed by purifying during growth to only allow certain nuclear-spin-free isotopes. Showing a coherent superconducting-semiconducting hybrid state in such a material raises hopes that future technology can exploit the capabilities of both types of materials.
“Superconducting coupling was very quickly apparent”
When developing their process, Scappucci group’s made devices with dimensions of hundreds of micrometers. As part of the collaboration, Chatterjee and her group decided to explore if their results held for smaller devices. PhD student Lazar Lakic fabricated devices showing that the process allowed for superconducting structures as small as 40 nanometers.
Along with postdoc William I. L. Lawrie, Lakic demonstrated that additionally, these could couple coherently to the quantum dots used for spin qubit implementations. Chatterjee recollects: “The process discovered by Giordano Scappucci’s group was immediately exciting to us; we spotted that it could enable us to design almost any circuit we could think of, by patterning top-down and annealing.”
The researchers subsequently designed a device with superconducting leads near a semiconducting quantum dot. Unexpectedly, the signature of the superconducting coupled bound state leaped out clearly (called a Yu-Shiba-Rusinov state after the theorists who discovered it).
Future prospects of potentially groundbreaking materials
The success of the device has made Chatterjee eager to work on future steps: “Along with the Scappucci group, we would like to start exploring other materials to try in this same annealing process. We used platinum for our study, which had the advantage of demonstrating some magnetic field resistance. But other metals exist that may potentially also form a superconducting alloy, such as niobium.”
Incorporating superconducting alloys made from different metals into a semiconductor allows for investigation of new emerging physics, for example the effects of unconventional superconductivity. Lawrie further explains: “For now, using this technique, we’re looking forward to developing new quantum coherent devices, and eventually building heterogeneous quantum processors that use the strengths of each qubit platform in the best way.”